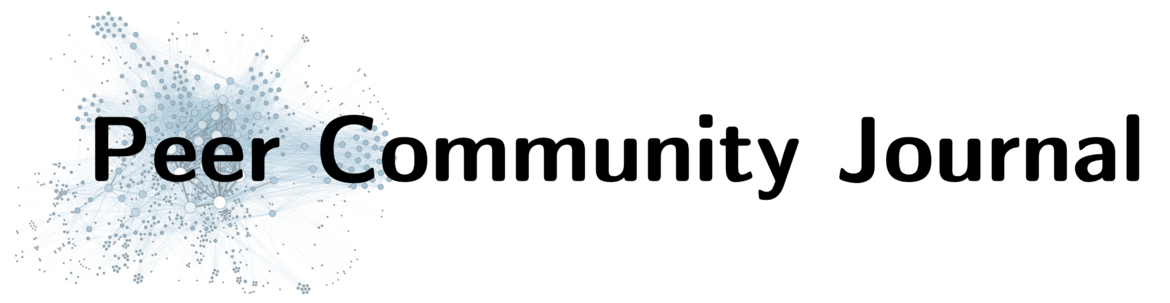
Section: Animal Science
Topic:
Agricultural sciences,
Applied biological sciences
Modelling the impact of the macroalgae Asparagopsis taxiformis on rumen microbial fermentation and methane production
Corresponding author(s): Muñoz-Tamayo, Rafael (Rafael.munoz-tamayo@inrae.fr)
10.24072/pcjournal.11 - Peer Community Journal, Volume 1 (2021), article no. e7.
Get full text PDF Peer reviewed and recommended by PCIBackground: the red macroalgae Asparagopsis taxiformis is a potent natural supplement for reducing methane production from cattle. A. taxiformis contains several anti-methanogenic compounds including bromoform that inhibits directly methanogenesis. The positive and adverse effects of A. taxiformis on the rumen microbiota are dose-dependent and operate in a dynamic fashion. It is therefore key to characterize the dynamic response of the rumen microbial fermentation for identifying optimal conditions on the use of A. taxiformis as a dietary supplement for methane mitigation. Accordingly, the objective of this work was to model the effect of A. taxiformis supplementation on the rumen microbial fermentation under in vitro conditions. We adapted a published mathematical model of rumen microbial fermentation to account for A. taxiformis supplementation. We modelled the impact of A. taxiformis on the fermentation and methane production by two mechanisms, namely (i) direct inhibition of the growth rate of methanogens by bromoform and (ii) hydrogen control on sugars utilization and on the flux allocation towards volatile fatty acids production. We calibrated our model using a multi-experiment estimation approach that integrated experimental data with six macroalgae supplementation levels from a published in vitro study assessing the dose-response impact of A. taxiformis on rumen fermentation. Results: our model captured satisfactorily the effect of A. taxiformis on the dynamic profile of rumen microbial fermentation for the six supplementation levels of A. taxiformis with an average determination coefficient of 0.88 and an average coefficient of variation of the root mean squared error of 15.2% for acetate, butyrate, propionate, ammonia and methane. Conclusions: our results indicated the potential of our model as prediction tool for assessing the impact of additives such as seaweeds on the rumen microbial fermentation and methane production in vitro. Additional dynamic data on hydrogen and bromoform are required to validate our model structure and look for model structure improvements. We expect this model development can be useful to help the design of sustainable nutritional strategies promoting healthy rumen function and low environmental footprint.
Type: Research article
Muñoz-Tamayo, Rafael 1; Chagas, Juana C. 2; Ramin, Mohammad 2; Krizsan, Sophie J. 2
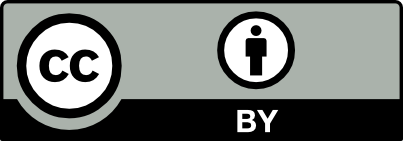
@article{10_24072_pcjournal_11, author = {Mu\~noz-Tamayo, Rafael and Chagas, Juana C. and Ramin, Mohammad and Krizsan, Sophie J.}, title = {Modelling the impact of the macroalgae {\protect\emph{Asparagopsis} taxiformis} on rumen microbial fermentation and methane production}, journal = {Peer Community Journal}, eid = {e7}, publisher = {Peer Community In}, volume = {1}, year = {2021}, doi = {10.24072/pcjournal.11}, language = {en}, url = {https://peercommunityjournal.org/articles/10.24072/pcjournal.11/} }
TY - JOUR AU - Muñoz-Tamayo, Rafael AU - Chagas, Juana C. AU - Ramin, Mohammad AU - Krizsan, Sophie J. TI - Modelling the impact of the macroalgae Asparagopsis taxiformis on rumen microbial fermentation and methane production JO - Peer Community Journal PY - 2021 VL - 1 PB - Peer Community In UR - https://peercommunityjournal.org/articles/10.24072/pcjournal.11/ DO - 10.24072/pcjournal.11 LA - en ID - 10_24072_pcjournal_11 ER -
%0 Journal Article %A Muñoz-Tamayo, Rafael %A Chagas, Juana C. %A Ramin, Mohammad %A Krizsan, Sophie J. %T Modelling the impact of the macroalgae Asparagopsis taxiformis on rumen microbial fermentation and methane production %J Peer Community Journal %D 2021 %V 1 %I Peer Community In %U https://peercommunityjournal.org/articles/10.24072/pcjournal.11/ %R 10.24072/pcjournal.11 %G en %F 10_24072_pcjournal_11
Muñoz-Tamayo, R.; Chagas, J. C.; Ramin, M.; Krizsan, S. J. Modelling the impact of the macroalgae Asparagopsis taxiformis on rumen microbial fermentation and methane production. Peer Community Journal, Volume 1 (2021), article no. e7. https://doi.org/10.24072/pcjournal.11
PCI peer reviews and recommendation, and links to data, scripts, code and supplementary information: 10.24072/pci.animsci.100006
Conflict of interest of the recommender and peer reviewers:
The recommender in charge of the evaluation of the article and the reviewers declared that they have no conflict of interest (as defined in the code of conduct of PCI) with the authors or with the content of the article.
[1] Review: Fifty years of research on rumen methanogenesis: lessons learned and future challenges for mitigation, Animal, Volume 14 (2020) no. 51 | DOI
[2] In Vitro Evaluation of Different Dietary Methane Mitigation Strategies, Animals, Volume 9 (2019) no. 12 | DOI
[3] Manipulating Rumen Fermentation, Journal of Animal Science, Volume 45 (1977) no. 3, pp. 585-599 | DOI
[4] Dynamic modelling of a single-stage high-rate anaerobic reactor—I. Model derivation, Water Research, Volume 25 (1991) no. 7, pp. 847-858 | DOI
[5] New inhibitors of methane production by rumen micro-organisms. Development and testing of inhibitors in vitro, British Journal of Nutrition, Volume 34 (2007) no. 3, pp. 429-446 | DOI
[6] Quantitation and diversity analysis of ruminal methanogenic populations in response to the antimethanogenic compound bromochloromethane, FEMS Microbiology Ecology, Volume 62 (2007) no. 3, pp. 313-322 | DOI
[7] Simulation of Nutrient Digestion, Absorption and Outflow in the Rumen: Model Description, The Journal of Nutrition, Volume 122 (1992) no. 11, pp. 2239-2256 | DOI
[8] Effect of Tropical Algae as Additives on Rumen <i>in Vitro</i> Gas Production and Fermentation Characteristics, American Journal of Plant Sciences, Volume 04 (2013) no. 12, pp. 34-43 | DOI
[9] Mode of action uncovered for the specific reduction of methane emissions from ruminants by the small molecule 3-nitrooxypropanol, Proceedings of the National Academy of Sciences, Volume 113 (2016) no. 22, pp. 6172-6177 | DOI
[10] MEIGO: an open-source software suite based on metaheuristics for global optimization in systems biology and bioinformatics, BMC Bioinformatics, Volume 15 (2014) no. 1 | DOI
[11] An evolutionary method for complex-process optimization, Computers & Operations Research, Volume 37 (2010) no. 2, pp. 315-324 | DOI
[12] Effect of high-sugar grasses on methane emissions simulated using a dynamic model, Journal of Dairy Science, Volume 95 (2012) no. 1, pp. 272-285 | DOI
[13] Seaweeds for animal production use, Journal of Applied Phycology, Volume 26 (2014) no. 2, pp. 891-899 | DOI
[14] Evaluation of predictions of volatile fatty acid production rates by the Molly cow model, Journal of Dairy Science, Volume 97 (2014) no. 1, pp. 354-362 | DOI
[15] Regulation mechanisms in mixed and pure culture microbial fermentation, Biotechnology and Bioengineering, Volume 111 (2014) no. 11, pp. 2139-2154 | DOI
[16] An inhibitor persistently decreased enteric methane emission from dairy cows with no negative effect on milk production, Proceedings of the National Academy of Sciences, Volume 112 (2015) no. 34, pp. 10663-10668 | DOI
[17] Addressing Global Ruminant Agricultural Challenges Through Understanding the Rumen Microbiome: Past, Present, and Future, Frontiers in Microbiology, Volume 9 (2018) | DOI
[18] Influence of hydrogen on rumen methane formation and fermentation balances through microbial growth kinetics and fermentation thermodynamics, Animal Feed Science and Technology, Volume 160 (2010) no. 1-2, pp. 1-22 | DOI
[19] microPop: Modelling microbial populations and communities in R, Methods in Ecology and Evolution, Volume 9 (2018) no. 2, pp. 399-409 | DOI
[20] Mitigating the carbon footprint and improving productivity of ruminant livestock agriculture using a red seaweed, Journal of Cleaner Production, Volume 259 (2020) | DOI
[21] The red macroalgae Asparagopsis taxiformis is a potent natural antimethanogenic that reduces methane production during in vitro fermentation with rumen fluid, Animal Production Science, Volume 56 (2016) no. 3, pp. 282-289 | DOI
[22] Asparagopsis taxiformis decreases enteric methane production from sheep, Animal Production Science, Volume 58 (2016) no. 4, pp. 681-688 | DOI
[23] A Concordance Correlation Coefficient to Evaluate Reproducibility, Biometrics, Volume 45 (1989) no. 1, pp. 255-268 | DOI
[24] Bayesian mechanistic modeling of thermodynamically controlled volatile fatty acid, hydrogen and methane production in the bovine rumen, Journal of Theoretical Biology, Volume 480 no. 2019, pp. 150-165 | DOI
[25] Dose-response effects of Asparagopsis taxiformis and Oedogonium sp. on in vitro fermentation and methane production, Journal of Applied Phycology, Volume 28 (2016) no. 2, pp. 1443-1452 | DOI
[26] Identification of bioactives from the red seaweed Asparagopsis taxiformis that promote antimethanogenic activity in vitro, Journal of Applied Phycology, Volume 28 (2016) no. 5, pp. 3117-3126 | DOI
[27] Effects of Marine and Freshwater Macroalgae on In Vitro Total Gas and Methane Production, PLoS ONE, Volume 9 (2014) no. 1 | DOI
[28] In Vitro Response of Rumen Microbiota to the Antimethanogenic Red Macroalga Asparagopsis taxiformis, Microbial Ecology, Volume 75 (2018) no. 3, pp. 811-818 | DOI
[29] The Potential Role of Seaweeds in the Natural Manipulation of Rumen Fermentation and Methane Production, Scientific Reports, Volume 6 (2016) no. 1 | DOI
[30] Seaweeds for livestock diets: A review, Animal Feed Science and Technology, Volume 212 (2016), pp. 1-17 | DOI
[31] Mathematical Modelling of the Anaerobic Digestion Process: Regulatory Mechanisms for the Formation of Short-Chain Volatile Acids from Glucose, Water Science and Technology, Volume 15 (1983) no. 8-9, pp. 209-232 | DOI
[32] Mechanistic modelling of in vitro fermentation and methane production by rumen microbiota, Animal Feed Science and Technology, Volume 220 (2016), pp. 1-21 | DOI
[33] IDEAS: a Parameter Identification Toolbox with Symbolic Analysis of Uncertainty and its Application to Biological Modelling, IFAC Proceedings Volumes, Volume 42 (2009) no. 10, pp. 1271-1276 | DOI
[34] Hydrogenotrophic methanogens of the mammalian gut: Functionally similar, thermodynamically different—A modelling approach, PLOS ONE (2019), Volume 14 (2019) no. 12 | DOI
[35] Review: To be or not to be an identifiable model. Is this a relevant question in animal science modelling?, Animal, Volume 12 (2018) no. 4, pp. 701-712 | DOI
[36] Thermodynamic modeling of ruminal fermentations, Animal Research, Volume 55 (2006) no. 5, pp. 343-365 | DOI
[37] Chemical defence against bacteria in the red alga Asparagopsis armata: linking structure with function, Marine Ecology Progress Series, Volume 306 (2006), pp. 87-101 | DOI
[38] Cellulose fermentation by continuous cultures of Ruminococcus albus and Methanobrevibacter smithii, Applied Microbiology and Biotechnology, Volume 33 (1990) no. 1, pp. 109-116 | DOI
[39] Effects of replacing rapeseed meal with fava bean at 2 concentrate crude protein levels on feed intake, nutrient digestion, and milk production in cows fed grass silage–based diets, Journal of Dairy Science, Volume 99 (2016) no. 10, pp. 7993-8006 | DOI
[40] Development of an in vitro method for determination of methane production kinetics using a fully automated in vitro gas system—A modelling approach, Animal Feed Science and Technology, Volume 174 (2012) no. 3-4, pp. 190-200 | DOI
[41] Effect of the macroalgae Asparagopsis taxiformis on methane production and rumen microbiome assemblage, Animal Microbiome, Volume 1 (2019) | DOI
[42] Inclusion of Asparagopsis armata in lactating dairy cows’ diet reduces enteric methane emission by over 50 percent, Journal of Cleaner Production, Volume 234 (2019), pp. 132-138 | DOI
[43] Red seaweed (Asparagopsis taxiformis) supplementation reduces enteric methane by over 80 percent in beef steers, PLOS ONE, Volume 16 (2021) no. 3 | DOI
[44] Reassessment of Biases in Predicted Nitrogen Flows to the Duodenum by NRC 2001, Journal of Dairy Science, Volume 86 (2003) no. 1, pp. 344-350 | DOI
[45] Effects of the macroalga Asparagopsis taxiformis and oregano leaves on methane emission, rumen fermentation, and lactational performance of dairy cows, Journal of Dairy Science, Volume 104 (2021) no. 4, pp. 4157-4173 | DOI
[46] Metabolic Hydrogen Flows in Rumen Fermentation: Principles and Possibilities of Interventions, Frontiers in Microbiology, Volume 11 (2020) | DOI
[47] Practical identifiability of a biokinetic model of activated sludge respiration, Water Research, Volume 29 (1995) no. 11, pp. 2561-2570 | DOI
[48] On the identifiability and distinguishability of nonlinear parametric models, Mathematics and Computers in Simulation, Volume 42 (1996) no. 2-3, pp. 125-134 | DOI
[49] Effects of phlorotannins from Ascophyllum nodosum (brown seaweed) on in vitro ruminal digestion of mixed forage or barley grain, Animal Feed Science and Technology, Volume 145 (2008) no. 1-4, pp. 375-395 | DOI
[50] Reaction of multihalogenated hydrocarbons with free and bound reduced vitamin B12, Biochemistry, Volume 7 (1968) no. 5, pp. 1707-1713 | DOI
Cited by Sources: